Dielectric and conductivity spectroscopy
By combining different experimental set-ups we track the dynamics of solids and solid-like materials in the enormous range of about 15 orders of magnitude. In addition we can vary the sample temperature continuously in a range from 4 to more than 500 K. This allows us to study the reorientation of molecular dipole moments and the transport of ionic charges over wide intervals. The excitation of the sample is by voltage steps, harmonic fields, as well as by electrical noise. Our main interest is to study the temperature dependence of rotational and translational correlation times as well as their distributions in order to gain microscopic insights into motional process in condensed matter.
Selected references
- R. Böhmer, B. Schiener, J. Hemberger, R. V. Chamberlin, Pulsed dielectric spectroscopy of supercooled liquids, Z. Phys. B - Condensed Matter 99, 91-99 (1995); 99, 624 (1996)
- A. Pimenov, P. Lunkenheimer, H. Rall, R. Kohlhaas, A. Loidl, R. Böhmer, Ion transport in the fragile glass former 3KNO3-2Ca(NO3)2, Phys. Rev. E 54, 676-684 (1996)
- O. Kircher, R. Böhmer, G. Hinze, Pseudo-stochastic multiple-pulse excitation in dielectric spectroscopy: Application to a relaxor ferroelectric, J. Phys. - Condensed Matter 15, S1069-S1075 (2003)
- C. Gainaru, R. Böhmer, G. Williams, Ion-sweeping in conducting dielectric materials, Eur. Phys. J. B 75, 209-216 (2010)
|
 |
Nonresonant dielectric hole burning
Most of the experiments in our laboratory are performed in the linear regime in which the polarization response is proportional to the electrical excitation. In addition, we developed and applied non-linear techniques such as nonresonant dielectric hole burning. This pump and probe technique is able to distinguish homogeneous from heterogeneous scenarios of relaxation. Developed together with Ralph V. Chamberlin from Arizona State University, this pump, wait, and probe experiment is able to reveal whether the broadening of a dielectric loss spectrum is due to a distribution of relaxation times or not. In the latter case the spectrum can be modified by a frequency selective pump employing very strong electrical fields with amplitudes in the kilovolt range. The altered correlation function is probed as the response to a small voltage step. The detection of the modified differential response is based on the shown phase cycle. This experiment can be considered as the low-frequency variant of related optical or nuclear-magnetic hole burning techniques. In combination with appropriate theoretical modeling this experiment has been successful in clarifying the nature of various relaxation processes in systems as diverse as supercooled liquids, relaxor ferroelectrics, orientationally disordered crystals, and ionic conductors, to name a few.
Selected references
- B. Schiener, R. Böhmer, A. Loidl, R. V. Chamberlin, Nonresonant spectral hole burning in the slow dielectric response of supercooled liquids, Science 274, 752-754 (1996)
- R. Richert, R. Böhmer, Heterogeneous and homogeneous diffusivity in an ion conducting glass, Phys. Rev. Lett. 83, 4337-4340 (1999)
- R. Böhmer, G. Diezemann, Principles and applications of pulsed dielectric spectroscopy and nonresonant dielectric hole burning, in: Broadband dielectric spectroscopy, edited by F. Kremer and A. Schönhals (Springer, Berlin, 2002), p. 523-569
|
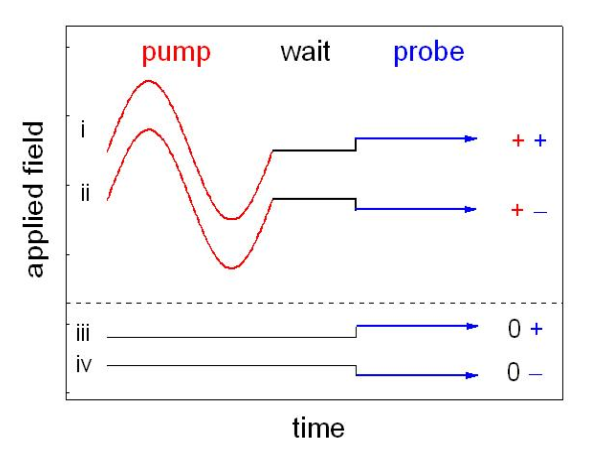 |
Solid-state nuclear magnetic resonance
An array of NMR techniques, embracing spin-lattice relaxometry, line shape analysis, two-dimensional exchange spectroscopy, and field-gradient measurements is used in our laboratory to study the rotational and translational motions in solids and solid-like samples. This gives us access to a wide range of time scales which we study in a temperature range extending from 4 to 500 K. We are equipped with several dedicated high-power and commercial spectrometers.
Selected references
- R. Böhmer, G. Diezemann, G. Hinze, E. Rössler, Dynamics of supercooled liquids and glassy solids, Prog. NMR Spectrosc. 39, 191-267 (2001)
- R. Böhmer, K. R. Jeffrey, M. Vogel, Solid-state lithium NMR with applications to the translational dynamics in ion conductors, Prog. NMR Spectrosc. 50, 87-174 (2007)
Multiple-time NMR correlation functions
Using the stimulated echo which can be pulse sequence of a radio-frequency pulse we study the slow dynamics, mostly via quadrupolar nucleus deuterium and lithium as probes. Hence we can generate signals of the type

- Recorded as a function of the mixing time tm the two-time correlation function F2 provides direct access to motional times scales.
- At a fixed mixing time this 3-pulse sequence can often be regarded as a low-pass filter which selects molecules or ions which did not move during tm. Using a fourth pulse the magnetization can be stored for latter use.
- The two-time correlation of the selected subensemble is tested via the G4(tm3) function.
- Another four-time correlation function is obtained by using the same low-pass filter twice. It enables us to detect whether the correlation time of a molecule or ion changes during the time interval tm2 and has given valuable information on dynamical heterogeneities in glass-forming materials and ion conductors.
Selected references
- G. Hinze, R. Böhmer, G. Diezemann, H. Sillescu, Experimental determination of four-time stimulated echoes in liquids, colloidal suspensions, and crystals, J. Magn. Reson. 131, 218-223 (1998)
- R. Böhmer, Multiple-time correlation functions from spin-3/2 solid-state NMR spectroscopy, J. Magn. Reson. 147, 78-88 (2000).
|
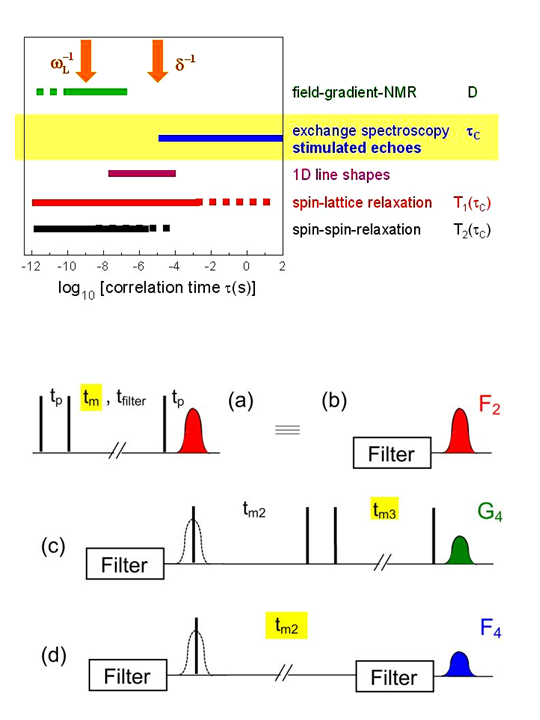 |
Near infrared spectroscopy
The spectral region beyond the long-wavelength end of visible light (near infrared: 3000 to 700 nm) can be exploited to study vibrations of OH, NH, and CH groups within liquids and solids. By investigating the intensities and frequencies of these vibrations access is provided to the relative number and the sizes of various hydrogen bonded associates for a wide temperature range. The consequences of diluting H-bonded networks with (non-) polar solvents and the resulting degree of hydrogen bonding can be monitored directly in the near-infrared region. Using time-resolved near-infrared experiments we map out slow H-bond exchange dynamics.
Selected references
- C. Gainaru, S. Kastner, F. Mayr, P. Lunkenheimer, S. Schildmann, H. J. Weber, W. Hiller, A. Loidl, R. Böhmer, Hydrogen-bond equilibria and life times in a monohydroxy alcohol, Phys. Rev. Lett. 107, 118304/1-5 (2011)
- M. Preuß, C. Gainaru, T. Hecksher, S. Bauer, J. C. Dyre, R. Richert, R. Böhmer, Experimental studies of Debye-like process and structural relaxation in mixtures of 2-ethyl-1-hexanol and 2-ethyl-1-hexyl bromide, J. Chem. Phys. 137, 144502/1-10 (2012)
|
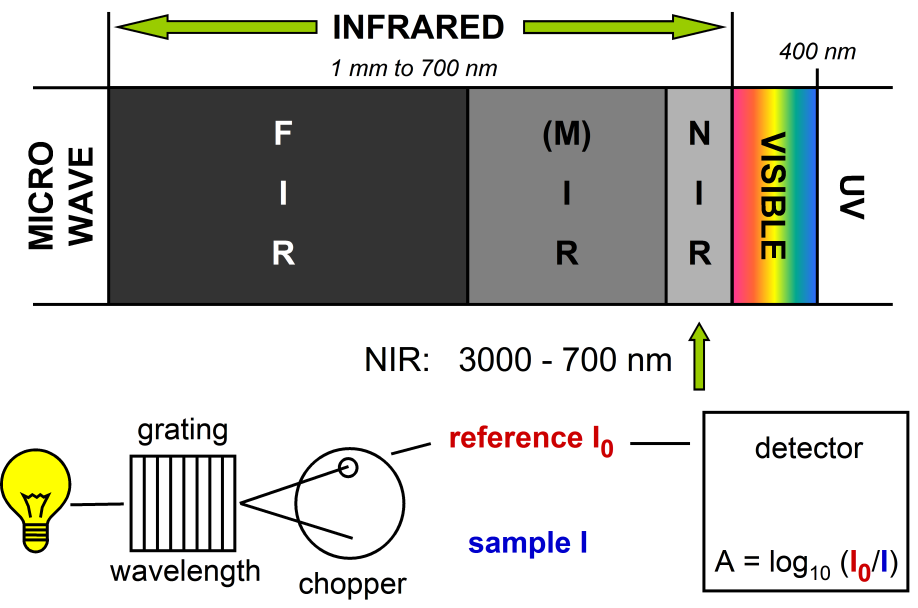 |